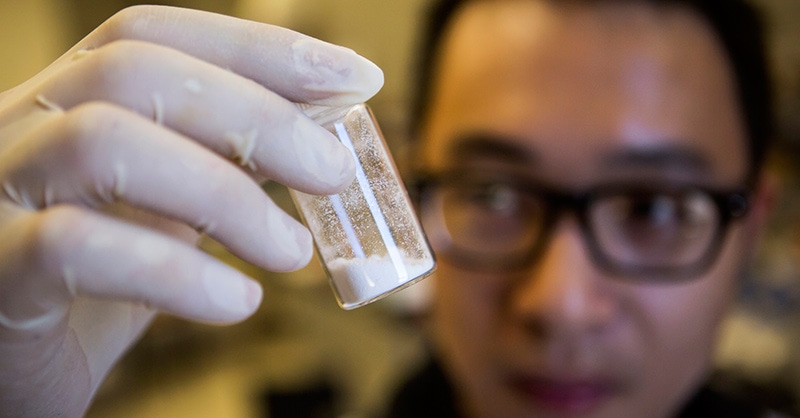
Regenerative medicine: Injectable stem cell incubator
By simulating the structure of the target tissue, the cells can better integrate with the body.
By simulating the structure of the target tissue, the cells can better integrate with the body.
By Kate McAlpine
Michigan Engineering
Some tissue damage is too extensive for the body to heal well, such as a bad slipped disc or the muscle death that follows a heart attack, so researchers are looking for ways to bridge the gaps. The most promising avenue is stem cell therapy, but these cells take their cues about what kind of cell to be from their surroundings.
Now, a team of researchers from the University of Michigan has developed microscopic particles that can be tailored for different parts of the body, loaded with stem cells, and injected straight into damaged tissues.
The new “microspheres” amount to an injectable kind of scaffolding that could hold the stem cells in place while they assumed their new roles, replacing dead tissue.
To make them, the researchers tweaked two aspects of the biodegradable molecules that make up the spheres, enabling the control of their solidity and fibrousness.
By simulating the structure of the target tissue, the cells can better integrate with the body, says Peter Ma, the Richard H. Kingery Collegiate Professor who led the development of the spheres.
“The degree of the hollowness and the smallness of the fibers that make up the microspheres can dramatically facilitate tissue or organ regeneration,” said Ma. “Currently, these novel microspheres are being used to regenerate intervertebral disc and heart tissues in our lab and our collaborators’ labs. If successful, we will potentially develop ways to cure low back pain and rescue patients suffering from heart attacks.”
His group previously showed that microspheres loaded with stem cells could help heal cartilage in the knees of rabbits when injected into the damaged area.
Ma’s team worked with the group of Sharon Glotzer, the Stuart W. Churchill Professor of Chemical Engineering, to discover a way to control the structure of the microspheres so that they can effectively support the repair of other types of tissue.
The microspheres are made of lactic acid, a product of metabolism, arranged into star-shaped polymers – so the human body can easily break them down. The team first mixed the polymers into glycerol, which is commonly used as sweetener. In the solution, the star-shaped polymers assembled into microspheres about 0.06 millimeters in diameter.
Then, the team tailored the nanoscale structure of the microspheres, which must mimic the network of proteins and other molecules that surround cells in the body, by plunging the mixture into liquid nitrogen at a temperature of about -321°F. If the lactic acid arms of the star-shaped polymers were too short, they formed solid microspheres. But if the arms were over about 150 to 200 lactic acids long, the polymers crystallized into fibers.
The trick to controlling the cell-scale porousness is in the hydroxyl groups, composed of a hydrogen atom and an oxygen atom stuck together, which are tacked onto the polymer. It doesn’t take many – less than one for every hundred lactic acid groups – but the hydroxyl allows the glycerol to support the microsphere structure. As a result, the polymer stars don’t feel the need to fill in every gap, leaving room for stem cells.
“Through manipulating the structure of star-shaped molecules, we can simultaneously control their assembly at both the nano- and microscales,” said Zhanpeng Zhang, who recently earned his doctorate in biomedical engineering, in part for his experimental work with the microspheres.
After washing out the glycerol, the team freeze-dried the microspheres for later use.
Computer simulations from Glotzer’s team confirmed that the interactions between the hydroxyl groups and the glycerol led to spongy or hollow the microspheres.
“Because the droplet assembly is so varied, we can also envision other applications such as drug delivery or catalysis, which in turn might require different kinds of nano- or micro-structure,” said Ryan Marson, who recently earned his doctorate in materials science and engineering, in part for his work on the simulations.
Other potential applications include electronic displays, self-healing materials and artificial photosynthesis.
Ma is also a professor of dentistry, biomedical engineering, macromolecular science and engineering and materials science and engineering. Glotzer is also a professor of materials science and engineering, macromolecular science and engineering, physics, and applied physics.
The research was supported by the National Institutes of Health, the Department of Defense, the National Science Foundation and the U.S. Army Research Office.