Summer Research
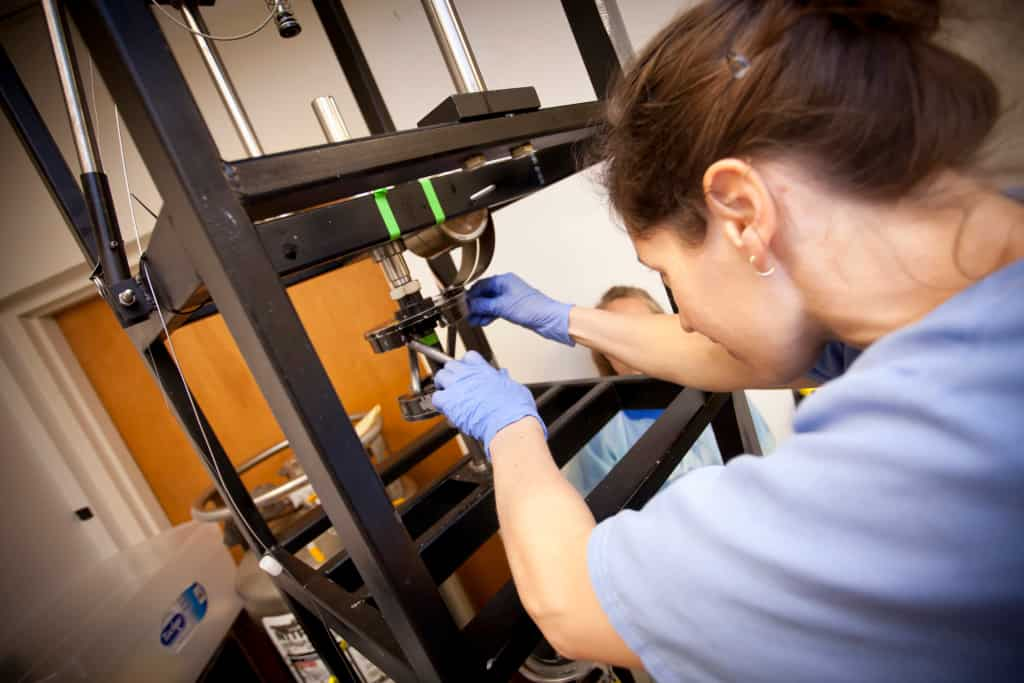
Make Your Summers Count
The Summer Undergraduate Research in Engineering (SURE) program provides summer research opportunities for U-M undergraduates; the Rackham Summer Research Opportunity Program (SROP) serves undergraduates from outside U-M.
Apply for a Summer Research Program
You are welcome to contact faculty if you have additional, specific questions regarding these projects. After your application is received (in late January), you will be contacted and asked to list your top three projects, in order of preference. You are also welcome to list these preferences on your application.
BME Guidelines:
Successful applicants will be selected by the projects’ listed faculty mentors. There is no requirement to contact the faculty mentor of your desired project(s) prior to being selected, but you may reach out to them with specific questions regarding the project if you desire. The number of positions awarded is dependent on SURE/SROP program allocations to the BME department (typically 6-8 each year).
Upcoming BME projects will be listed starting in November; the application period runs through late January.
Projects are added as they become available. Please check back for updated listings.
2024 BME Projects:
BME Project #1: Antibiotic resistance & drug combination discovery
Faculty Mentor: Sriram Chandrasekaran, Ph.D., csriram@umich.edu
Prerequisites: Familiarity with MATLAB programming. Basic knowledge of microbiology and genetics. Knowledge of machine learning is a plus.
Project Description: The focus of this project is to understand antibiotic resistance and design novel drug treatments. 100,000 people die and a million others are sickened by antibiotic resistant bacteria in the United States every year. There is an urgent need to develop high-throughput approaches to screen promising drugs to counter antibiotic-resistance. The student will apply computer algorithms developed in our lab to identify potent antibiotic combinations for treating drug resistant microbial infections.
Research Mode: Hybrid
BME Project #2: Cancer metabolism & precision medicine
Faculty Mentor: Sriram Chandrasekaran, Ph.D., csriram@umich.edu
Prerequisites: Familiarity with MATLAB or Python. Basic knowledge of biochemistry, molecular biology, and genetics. Experience working with big-data (genomics, transcriptomics) is a plus.
Project Description: This project involves the application of computer models to simulate the metabolic properties of tumors. The computer models will be built using genomics, metabolomics and transcriptomics data from various types of cancer cell lines. By understanding the unique metabolic properties of each cell type, we can design drugs that target specific tumors. Further, knowledge of these differences will be used to design synergistic drug combinations tailored to each patient.
Research Mode: Hybrid
BME Project #3: Guiding people with visual impairment with non-visual information
Faculty Mentor: James Weiland, PhD., weiland@umich.edu
Prerequisites: None.
Project Description: This project involves creating and testing novel wearable system to aid people with visual impairment. The research involves evaluating how best to provide non-visual information. The wearable system can give verbal and vibrotactile cues to orient then move towards a goal. The research will involve development of software and hardware.
Research Mode: In lab
BME Project #4: Engineering Wellness: A Qualitative Study to Support Undergraduate Engineering Student Well-Being Through Curriculum Development
Faculty Mentor: Karin Jensen, Ph.D., kjens@umich.edu
Prerequisites: None.
Project Description: This research explores the experiences of undergraduate engineering students with regards to stress and engineering culture. Specifically, this project focuses on the development and improvement of a required first-year engineering course (ENGR 100). Our instructional team created a BME-based section of this class that was piloted in the fall semester of 2023 as ENGR100.520: Engineering Wellness.
The main objective of ENGR 100 is to introduce first-year students to the design thinking process and help them learn how to communicate in a technical setting. With a focus on wellness, our section explores research and technologies that support both mental health and physical health. In addition to lectures, discussion, and lab, students work with a team to analyze and iterate on a commercially available at-home biosensor (e.g., Fitbit smartwatch, Garmin running watch, Muse meditation headband, etc.).
After our first semester teaching the course, we have many ideas for improving future implementations of section 520. To expand on these thoughts and identify new ideas, we plan to conduct focus groups with first-year engineering students and one-on-one interviews with undergraduate engineers at the University of Michigan. We will analyze this data to better understand student perceptions and experiences with an engineering culture of stress. Next, we will synthesize our results with prior research to determine aspects of wellness that faculty and staff can support in the classroom. The findings of this analysis will be applied in future implementations of ENGR100.520.
All interviews and focus groups (called just “interviews” from here forward) will be conducted by the doctoral candidate mentor. The SURE student will transcribe these interviews and keep notes or other related memos for the mentor. They will also help their mentor analyze interviews through organization of qualitative data and assistance with creating a codebook for transcripts. We expect to use thematic analysis during this process. The SURE student will be responsible for communicating progress in both verbal and written formats to the graduate student mentor. Finally, we hope to improve student wellness in the engineering classroom (ENGR100.520) through curriculum updates, policy change, culture shifts, and other implementations of our results.
Research Mode: In person (in the lab); Remote/Online, Hybrid
BME Project #5: Modulating myeloid cell phenotypes with immunomodulatory nanoparticles
Faculty Mentor: Lonnie Shea, Ph.D., ldshea@umich.edu
Prerequisites: Willingness to assist with surgery, extensively handle mice, use needles, and perform dissection and necropsy procedures.
Project Description: Idiopathic pulmonary fibrosis (IPF) is a devastating lung disease with a <50% 5-year survival rate. It is characterized by progressive deposition of excessive extracellular matrix proteins in the lungs (i.e., scarring) due to a dysfunctional wound healing response. The cause of IPF remains unknown and there is no cure and few treatments that slow progression. A key mechanism of IPF disease is the accumulation of dysregulated lung macrophages that promote fibrosis. While healthy lung macrophages are seeded in the lung during embryonic development, IPF macrophages are largely derived from circulating monocytes. We know that depleting these disease-causing macrophages from the lung can treat IPF in mice. However, macrophage depletion is not a viable strategy in the clinic because it causes significant side effects, especially dangerous immunosuppression. Previously, we developed immunomodulatory nanoparticles that are preferentially phagocytosed (internalized) by circulating monocytes and macrophages in the bloodstream. The particles cause immune cells to reduce inflammatory behaviors and acquire a pro-regenerative function instead. We have previously demonstrated that in cancer, these particles can reduce tumor size and prevent metastasis in mice. In spinal cord injury, the particles can improve mobility of injured mice by reducing inflammation. In IPF mouse models, we hypothesize that the particles will be phanocytosed by circulating IPF monocytes and prevent them from turning into fibrosis-causing macrophages in the lungs. Preliminary data suggests the particles reduce lung fibrosis in mice, supporting our hypothesis. This project is the first application of our nanoparticles in lung injury and addresses an urgent clinical need for improved IPF therapies.
Research Mode: In person (in the lab)
BME Project #6: Biomimetic Apoptotic Particles for Macrophage-driven Bone Regeneration
Faculty Mentor: Brendon M. Baker, Ph.D., bambren@umich.edu
Prerequisites: General lab experience, lab notebooking, cell and tissue culture, familiarity with MATLAB.
Project Description: Improper osseous wound healing due to disease, injury related trauma, and tumor resection, among other causes, can lead to impaired function, pain, reduced quality of life, and substantial costs to individuals. Often overlooked, one of the first steps in bone wound repair is cell death and subsequent apoptotic (dead/dying) cell clearance, called efferocytosis, by macrophages. In this project, a biomaterial-based imitation of efferocytosis will be investigated as a promising strategy to modulate and enhance bone regeneration. Students involved in this project will gain expertise in tissue engineering, including mammalian cell culture, biomaterials, and biological image analysis.
Research Mode: In lab
BME Project #7: Synthetic biomaterials to direct therapeutic angiogenesis
Faculty Mentor: Brendon M. Baker, Ph.D., bambren@umich.edu
Prerequisites: General lab experience, lab notebooking, cell and tissue culture, familiarity with MATLAB.
Project Description: Angiogenesis is a complex morphogenetic process that involves intimate interactions between migrating multicellular endothelial structures and their extracellular milieu. To investigate how microenvironmental cues regulate angiogenesis, we develop in vitro organotypic models that reduce the complexity of the native microenvironment and enable mechanistic insight into how soluble and physical extracellular matrix cues regulate this dynamic process. The focus of this project is to build a synthetic material that promotes angiogenesis without the need for exogenous soluble cues or growth factor gradients. This implantable biomaterial in the longer term will be applied to disease or injury settings to restore vascular function or for the creation of vascularized tissue grafts. Students involved in this project will gain expertise in biomaterials, microphysiologic modeling, and biological image analysis.
Research Mode: In lab
BME Project #8: Immuno-metabolic Sensor for Detection of Type1 Diabetes
Faculty Mentor: Lonnie Shea, Ph.D., ldshea@umich.edu
Prerequisites: Coding background in R/Python/MATLAB or strong wet lab experience.
Project Description: The goal of the project is to develop a immuno-metabolic sensor which can predict the onset of diabetes early enough for therapeutic intervention. We are using an implantable biomaterial scaffold made of poly-caprolactone as a sensor for Type 1 Diabetes onset. This involves in-vivo animal work where the scaffold is implanted subcutaneously and then explanted at different timepoints during the course of onset of Type 1 Diabetes. Single cell Sequencing and metabolomics of the scaffolds will be performed and then computational techniques will be used to detect immune cell and metabolite related biomarker for early prediction of onset of Type 1 Diabetes.
Research Mode: In person (in the lab)
BME Project #9: Building Synthetic Receptors To Detect Disease
Faculty Mentor: Aaron Morris, Ph.D., aharmorr@umich.edu
Prerequisites: None.
Project Description: Synthetic receptor systems are transforming healthcare in a multitude of ways, including development of cancer therapeutics (CAR-T cells) and treating infectious disease. Synthetic receptors allow scientists to modify cells with novel, bioorthogonal functionality and enable us to create cells that recognize new targets. This project will focus on making these receptors using molecular cloning techniques and integrating these receptors into cells. Techniques we will use include: cell culture, DNA purification, gene delivery, molecular cloning, and flow cytometry.
Research Mode: In person (In the lab)